General Overview
For my experiments, I used uBlox NEO M6, M7 and M8 modules. The modules were connected to a Laptop using a FT232RL-based UART to USB converter. Using the uBlox u-center software, the Timepulse 5 settings were adjusted to generate 10 kHz and 100 kHz signals with a duty cycle of 50 %. The generated commands were observed using the binary console view of the u-center software.
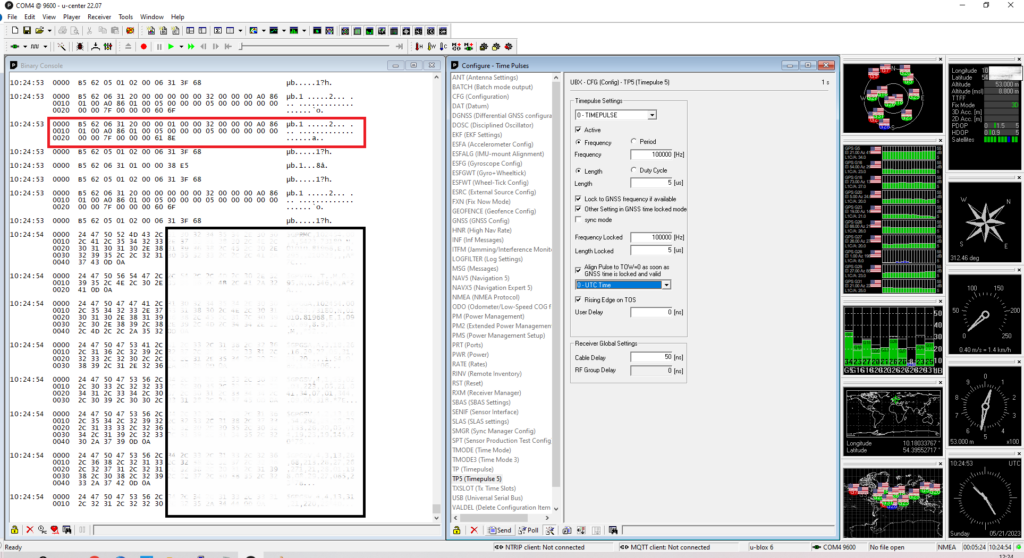
Setting the Timepulse 5 settings using the uBlox u-center software. Commands sent to the NEO-6 GPS module can be observed in the binary console view.
Those binary commands were then translated to C++ compatible arrays. These commands can now be sent to the GPS-modules using an Arduino quite easily. For instance, the command array to set Timepulse 5 to generate a 10 kHz reference signal with 50 % duty cycle, synchronized to UTC time if the receiver has a valid position fix is as follows:
// UBX CFG-TP5: Set 10 kHz, 50 uS Pulses (50 % duty-cycle), Synch UTC-Time, no output if GPS unlocked
byte TP5_10K_LOCK[]{0xB5, 0x62, 0x06, 0x31, 0x20, 0x00, 0x00, 0x01, 0x00, 0x00, 0x32, 0x00, 0x00, 0x00, 0x01, 0x00, 0x00, 0x00, 0x10, 0x27, 0x00, 0x00, 0x00, 0x00, 0x00, 0x00, 0x32, 0x00, 0x00, 0x00, 0x00, 0x00, 0x00, 0x00, 0x7F, 0x00, 0x00, 0x00, 0x73, 0xF3};
Sending this command to the GPS-module using the inbuilt UART can be accomplished as follows:
Serial.write(TP5_10K_LOCK, sizeof(TP5_10K_LOCK));
Another useful setting for GPS-locked frequency references is the CFG-NAV5 setting. It can be used to set the navigation mode to stationary.
// CFG-NAV5: Set navigation mode to stationary
byte CFG_NAV5_STATIONARY[] = { 0xB5, 0x62, 0x06, 0x24, 0x24, 0x00, 0xFF, 0xFF, 0x02, 0x03, 0x00, 0x00, 0x00, 0x00, 0x10, 0x27, 0x00, 0x00, 0x05, 0x00, 0xFA, 0x00, 0xFA, 0x00, 0x64, 0x00, 0x2C, 0x01, 0x00, 0x3C, 0x00, 0x00, 0x00, 0x00, 0x00, 0x00, 0x00, 0x00, 0x00, 0x00, 0x00, 0x00, 0x4E, 0x60 };
Note that all settings, including Timepulse 5 and CFG-NAV5, are volatile. That means the settings are lost upon power-cycling the module. This can be avoided by permanently storing the adjusted settings using the CFG-CFG-command.
// UBX CFG-CFG: Save all. BBR + FLASH:
byte CFG[] = {0xB5, 0x62, 0x06, 0x09, 0x0D, 0x00, 0x00, 0x00, 0x00, 0x00, 0xFF, 0xFF, 0x00, 0x00, 0x00, 0x00, 0x00, 0x00, 0x03, 0x1D, 0xAB };
After sending the CFG-CFG-command, all settings will be permanently stored and retrieved upon power-up. Therefore, a microcontroller is not necessary for operating a uBlox GPS-module as frequency reference. The settings can be adjusted dynamically during operation in order to generate different reference frequencies as needed.
Test Results
A simple and straight-forward Arduino sketch was written to test the commands on various uBlox modules. The code sets the Timepulse 5 output frequency to 10 kHz, the navigation mode to stationary and stores the settings permanently. It then goes on to change between 10 kHz and 100 kHz every 10 seconds. The Timepulse output was connected to an oscilloscope and the resulting waveforms were observed. As shown in the images below, the module successfully accepted the commands and alternatingly put out 10 kHz and 100 kHz signals when locked to a valid GPS-source.
Commands
Here is a list of useful commands in array-form for the Arduino IDE:
// UBX CFG-CFG: Save all. BBR + FLASH:
byte CFG[] = { 0xB5, 0x62, 0x06, 0x09, 0x0D, 0x00, 0x00, 0x00, 0x00, 0x00, 0xFF, 0xFF, 0x00, 0x00, 0x00, 0x00, 0x00, 0x00, 0x03, 0x1D, 0xAB };
// CFG-NAV5: Set navigation mode to stationary
byte CFG_NAV5_STATIONARY[] = { 0xB5, 0x62, 0x06, 0x24, 0x24, 0x00, 0xFF, 0xFF, 0x02, 0x03, 0x00, 0x00, 0x00, 0x00, 0x10, 0x27, 0x00, 0x00, 0x05, 0x00, 0xFA, 0x00, 0xFA, 0x00, 0x64, 0x00, 0x2C, 0x01, 0x00, 0x3C, 0x00, 0x00, 0x00, 0x00, 0x00, 0x00, 0x00, 0x00, 0x00, 0x00, 0x00, 0x00, 0x4E, 0x60 };
// UBX CFG-TP5 Timepulse 5: 10 kHz, 50 uS (50 % duty cycle), synch to UTC Time, No Putput when unlocked
byte TP5_10K_LOCK[]{ 0xB5, 0x62, 0x06, 0x31, 0x20, 0x00, 0x00, 0x01, 0x00, 0x00, 0x32, 0x00, 0x00, 0x00, 0x01, 0x00, 0x00, 0x00, 0x10, 0x27, 0x00, 0x00, 0x00, 0x00, 0x00, 0x00, 0x32, 0x00, 0x00, 0x00, 0x00, 0x00, 0x00, 0x00, 0x7F, 0x00, 0x00, 0x00, 0x73, 0xF3 };
// Timepulse 5: 100 kHz, 5 uS (50 % duty cycle), synch to UTC Time, No Putput when unlocked
byte TP5_100K_LOCK[]{ 0xB5, 0x62, 0x06, 0x31, 0x20, 0x00, 0x00, 0x01, 0x00, 0x00, 0x32, 0x00, 0x00, 0x00, 0x01, 0x00, 0x00, 0x00, 0xA0, 0x86, 0x01, 0x00, 0x00, 0x00, 0x00, 0x00, 0x05, 0x00, 0x00, 0x00, 0x00, 0x00, 0x00, 0x00, 0x7F, 0x00, 0x00, 0x00, 0x36, 0x36 };
// UBX CFG-TP5 Timepulse 5: 10 kHz, 50 uS (50 % duty cycle), synch to UTC Time, free running 10 kHz if unlocked
byte TP5_10K[] = { 0xB5, 0x62, 0x06, 0x31, 0x20, 0x00, 0x00, 0x01, 0x00, 0x00, 0x32, 0x00, 0x00, 0x00, 0x10, 0x27, 0x00, 0x00, 0x10, 0x27, 0x00, 0x00, 0x32, 0x00, 0x00, 0x00, 0x32, 0x00, 0x00, 0x00, 0x00, 0x00, 0x00, 0x00, 0x7F, 0x00, 0x00, 0x00, 0xDB, 0xFC };
// UBX CFG-TP5 Timepulse 5: 100 kHz, 5 uS (50 % duty cycle), synch to UTC Time, free running 100 kHz if unlocked
byte TP5_100K[] = { 0xB5, 0x62, 0x06, 0x31, 0x20, 0x00, 0x00, 0x01, 0x00, 0x00, 0x32, 0x00, 0x00, 0x00, 0xA0, 0x86, 0x01, 0x00, 0xA0, 0x86, 0x01, 0x00, 0x05, 0x00, 0x00, 0x00, 0x05, 0x00, 0x00, 0x00, 0x00, 0x00, 0x00, 0x00, 0x7F, 0x00, 0x00, 0x00, 0x61, 0x8E };
Arduino Code
/*
* uBlox u-blox NEO-6M / NEO-7M
*
* Copyright (C) 2022 Westerhold, S. (AI5GW)
* ORCID: https://orcid.org/0000-0001-7965-3140
* Web (EN): https://baltic-lab.com
*
* This program is free software: you can redistribute it and/or modify
* it under the terms of the GNU General Public License as published by
* the Free Software Foundation, either version 3 of the License, or
* (at your option) any later version.
*/
// CFG-NAV5: Set navigation mode to stationary
byte CFG_NAV5_STATIONARY[] = { 0xB5, 0x62, 0x06, 0x24, 0x24, 0x00, 0xFF, 0xFF, 0x02, 0x03, 0x00, 0x00, 0x00, 0x00, 0x10, 0x27, 0x00, 0x00, 0x05, 0x00, 0xFA, 0x00, 0xFA, 0x00, 0x64, 0x00, 0x2C, 0x01, 0x00, 0x3C, 0x00, 0x00, 0x00, 0x00, 0x00, 0x00, 0x00, 0x00, 0x00, 0x00, 0x00, 0x00, 0x4E, 0x60 };
// UBX CFG-CFG: BBR + FLASH:
byte CFG[] = { 0xB5, 0x62, 0x06, 0x09, 0x0D, 0x00, 0x00, 0x00, 0x00, 0x00, 0xFF, 0xFF, 0x00, 0x00, 0x00, 0x00, 0x00, 0x00, 0x03, 0x1D, 0xAB };
// UBX CFG-TP5 Timepulse 5: 10 kHz, 50 uS (50 % duty cycle), synch to UTC Time, No Putput when unlocked
byte TP5_10K_LOCK[]{ 0xB5, 0x62, 0x06, 0x31, 0x20, 0x00, 0x00, 0x01, 0x00, 0x00, 0x32, 0x00, 0x00, 0x00, 0x01, 0x00, 0x00, 0x00, 0x10, 0x27, 0x00, 0x00, 0x00, 0x00, 0x00, 0x00, 0x32, 0x00, 0x00, 0x00, 0x00, 0x00, 0x00, 0x00, 0x7F, 0x00, 0x00, 0x00, 0x73, 0xF3 };
// Timepulse 5: 100 kHz, 5 uS (50 % duty cycle), synch to UTC Time, No Putput when unlocked
byte TP5_100K_LOCK[]{ 0xB5, 0x62, 0x06, 0x31, 0x20, 0x00, 0x00, 0x01, 0x00, 0x00, 0x32, 0x00, 0x00, 0x00, 0x01, 0x00, 0x00, 0x00, 0xA0, 0x86, 0x01, 0x00, 0x00, 0x00, 0x00, 0x00, 0x05, 0x00, 0x00, 0x00, 0x00, 0x00, 0x00, 0x00, 0x7F, 0x00, 0x00, 0x00, 0x36, 0x36 };
void setup() {
Serial.begin(9600);
// Set Timepulse output to 100 kHz, 50 % duty-cycle
Serial.write(TP5_10K_LOCK, sizeof(TP5_10K_LOCK));
// Set navigation mode to stationary
Serial.write(CFG_NAV5_STATIONARY, sizeof(CFG_NAV5_STATIONARY));
// Store settings permanently
Serial.write(CFG, sizeof(CFG));
}
// loop switches between 10 kHz and 100 kHz every 10 seconds
void loop() {
Serial.write(TP5_10K_LOCK, sizeof(TP5_10K_LOCK));
delay(10000);
Serial.write(TP5_100K_LOCK, sizeof(TP5_100K_LOCK));
delay(10000);
}